bone
Contributions of Resin Cast Etching to Visualising the Osteocyte Lacuno-Canalicular Network Architecture in Bone Biology and Tissue Engineering

The osteocyte lacuno-canalicular network is a fundamental structural and functional component of bone. The osteocytes play the vital role of mechanosensory cells by orchestrating bone remodeling. Furthermore, the morphology of the osteocyte lacuno-canalicular network (e.g., osteocyte interconnectivity) reflects the underlying mechanobiological status of bone, which is relevant for understanding the processes of bone growth and repair/renewal. To better characterize this complex morphology, novel high-resolution imaging techniques have been implemented, including tissue specimens from various types of implanted biomaterials. Of the various imaging techniques available, resin cast etching is a straightforward method involving sequential acid etching and alkali digestion of resin embedded bone to visualize the osteocyte lacuno-canalicular network using scanning electron microscopy.
This technique was first proposed in 1985, where Todd Curtis and co-workers used resin cast etching to first characterize trabecular and cortical bone morphology we all have come to know today. They did this by visualizing the osteocytes as flattened oval discs aligned parallel to the successive layers of collagen. Today, this technique has been applied across the spectrum of research, from developmental biology to the impact of genetic alterations on bone, ageing, spinal cord injury and long-term immobilization, to the effects of cancer on bone, tissue engineering, and finally, to drug discovery. Two unique applications of resin cast etching in the clinical and experimental biomaterials research field include: 1) visualizing the distribution of osteocytes around metal implants used in dentistry, craniomaxillofacial surgery, and orthopedics; and 2) determining the direction of bone formation around resorbable biomaterials designed to regenerate bone lost due to trauma or surgery (e.g., calcium phosphates). Resin cast etching has revealed the three-dimensional morphology of osteocytes and their lacuno-canalicular network, providing evidence of alterations in network interconnectivity due to changes in either osteocyte density—the number of osteocytes per unit area—or dendricity—the number of canaliculi per osteocyte lacuna. Furthermore, resin cast etching is a convenient way to characterize lacunar shape and size, which are important markers of development, aging, and bone regeneration. Ultimately, the quality of information gathered from the resin cast is dependent on sample preparation. In some situations (e.g., a heterogeneously dense specimen), sub-optimal infiltration and polymerization of the embedding medium results in areas of bone that falsely appear devoid of the osteocyte lacuno-canalicular network, and alternative imaging techniques will need to be considered.
Resin cast etching offers advantages over other techniques that are commonly used to examine the osteocyte lacuno-canalicular system. Most notably, any resin embedded specimen, such as clinical biopsies, can be used for resin cast etching without the need for additional contrast staining. The added convenience of performing additional assessments of bone mineral density distribution and elemental analysis—all within the same scanning electron microscope—make resin cast etching ideal for gathering both quantitative and qualitative information of the osteocyte morphology. One such approach in the bone repair biomaterials community has used resin cast etching to examine the bone response in novel biomaterials applications, including those biomaterials that gradually degrade in vivo and/or possess the capability to deliver substances that impact bone metabolism (e.g., bone morphogenetic proteins). Over time, these types of investigations are expected to stimulate the development and validation of advanced therapeutic strategies targeting pathological and rare bone diseases in addition to commonly encountered conditions such as osteoporosis.
The authors wish to thank the Japan Society for the Promotion of Science (JSPS; grant number 17K17564), Takeda Science Foundation, the Svenska Sällskapet för Medicinsk Forskning (SSMF), the Hjalmar Svensson Foundation, and the Kungliga Vetenskaps-och Vitterhets-Samhället i Göteborg.
Molecular Identification of Spatially Distinct Anabolic Responses to Mechanical Loading in Murine Cortical Bone
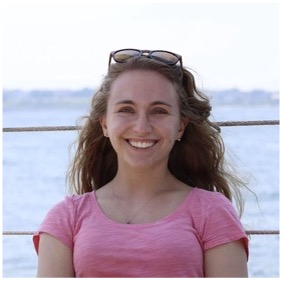
Meinig School of Biomedical Engineering, Cornell University, Ithaca, NY, USA
Osteoporosis is a bone disease that causes decreased bone quality and strength, resulting in increased fracture risk. Currently, anabolic, bone-forming therapies are limited, highlighting the need for novel therapeutic options. Mechanical loading of the skeleton produces anabolic tissue responses and increases bone mass clinically and in preclinical models. This anabolic tissue response is driven by a cascade of osteogenic signaling pathways, few of which have been identified, suggesting that more remain to be discovered. Transcriptomic profiles provide insight into biological pathways activated by mechanical loading. Tibial compression produces varying deformation magnitudes along the axial direction of the cortex, inducing the highest strains at the mid-diaphysis and the lowest at the metaphyseal shell. Anabolic pathways in the cortical bone that are differentially activated based on strain magnitude may identify promising novel therapeutic targets and have not been explored in previous transcriptomic studies.
In our study published in JBMR (2022), we sought to elucidate the role of mechanical strain magnitude on the transcriptional response of cortical bone during loading. The left limb of female mice was loaded in compression, and the right limb served as the contralateral control. Gene expression was evaluated at early time points following a single bout of loading (1 h, 3 h, and 24 h) or at 1 wk following daily bouts of loading. Taking advantage of the natural gradient of strain induced by cyclic tibial compression, we isolated RNA from three strain regions in the cortex: metaphyseal cortical shell (low strain), proximal diaphysis (medium strain), and mid-diaphysis (high strain). Differential gene expression was analyzed between loaded and control limbs, correlated with enrichment of biological processes, and validated with in situ hybridization. We found that transcriptomic responses correlated with tissue strain magnitude; at each time point, the mid-diaphysis (highest strain) had the most differentially-expressed genes and the metaphyseal cortical shell (lowest strain) had the least. Similarly, biological processes regulating bone formation and turnover increased earlier and to the greatest extent at the mid-diaphysis.
Higher strain induced greater levels of osteocyte-associated genes (Sost, Mepe), whereas expression was lower in osteoclast-related genes (Ctsk, Acp5). The differentially-expressed genes and biological processes were unique across all three tissue segments. Finally, the distinct transcriptomic responses recorded at each time point following loading highlight the complex cascade of osteogenic signaling induced by loading.
In summary, cortical bone responded to mechanical loading as a function of strain magnitude across early and late time points. In cortical bone, higher strain magnitudes elicited larger, earlier anabolic responses in cortical bone whereas low strain magnitude was correlated with a diminished response. This research highlights the importance of spatial evaluation of transcriptional profiles. Future work using bulk RNA sequencing should distinguish the cortical segment by axial location or mechanical strain experienced. Finally, this work enhanced our understanding of the role of mechanical strain in the transcriptomic response of cortical bone to loading, thereby improving the ability to create effective targeted therapeutics.
This work was conducted by Carolyn Chlebek, Jacob Moore, F. Patrick Ross, and Marjolein van der Meulen. Funding for this research was provided by NSF Grant #1636012, NSF GRFP (DGE-1650441), and GAANN P200A150273 J. The authors would like to thank Dr. Adrian McNairn and Dr. John Schimenti for their experimental assistance and for providing essential training that enabled this research. For assistance with analysis, the authors acknowledge the Cornell University Bioinformatics Facility and specifically Dr. Qi Sun. Danielle Jorgenson also assisted in data analysis and sorting. The authors also acknowledge the Cornell CARE staff. Transcriptomic data presented in this study are available in Gene Expression Omnibus, Accession GSE210827.